Transport in Nanocrystal Solids
Thermal Transport in 3D Nanocrystal Arrays
Arrays of ligand-stabilized colloidal nanocrystals with size-tunable electronic structure are promising alternatives to single-crystal semiconductors in electronic, optoelectronic and energy-related applications. Hard/soft interfaces—associated with the nanocrystal–ligand core–shell structure—in these nanocrystal arrays (NCAs) create a complex and uncharted vibrational landscape for thermal energy transport that will influence their technological feasibility.
We present thermal conductivity measurements of NCAs (CdSe, PbS, PbSe, PbTe, Fe3O4 and Au) and reveal that energy transport is mediated by the density and chemistry of the organic/inorganic interfaces, and the volume fractions of nanocrystal cores and surface ligands. NCA thermal conductivities are controllable within the range 0.1–0.3 W m−1 K−1, and only weakly depend on the thermal conductivity of the inorganic core material. This range is 1,000 times lower than the thermal conductivity of silicon, presenting challenges for heat dissipation in NCA-based electronics and photonics. It is, however, 10 times smaller than that of Bi2Te3, pointing to potential applications in NCA-based thermoelectric materials.

Thermal transport studies of various nanocrystals.
Composition-Matched Semiconductor Solders
Soldering and related processes are very useful in many areas, from microelectronics to plumbing. Metals can be easily connected both mechanically and electrically. Unlike the case for metals, there are no established methods for joining semiconductor pieces under mild conditions without disrupting semiconducting properties at the joint.
The electronic properties of semiconductor interfaces are much more sensitive to impurities and structural defects than are metals. Metal–metal contacts always show ohmic behavior, whereas semiconductor–semiconductor interfaces are more complex. Misalignment of the Fermi energy levels or trapping charge carriers at the interface creates a Schottky barrier.
The chalcogenidometallates have been successfully explored as soluble precursors for inorganic semiconductors. We for the first time demonstrated that various chalcogenidometallate complexes can serve as convenient ligands stabilizing colloidal nanocrystals and nanowires.
Upon gentle heat treatment, these ligands can be converted into semiconducting phases, generating inorganic nanocrystal solids. As a consequence, beyond colloidal stabilization, the compositional-matched ligands can be used as semiconductor solders. This concept has been successfully applied in various technologically important II-VI, IV-VI, and V-VI semiconductors, including CdSe, CdTe, HgxCd1−xTe, PbTe, (BixSb1−x)2Te3, and Bi2Te3.
Demonstration of a variety of chalcogenidometallate ligands colloidally stabilizing various nanocrystals.


Transport properties of CdSe NCs after ligand exchange.
The successful application of these new semiconductor solders was demonstrated in solution-processed high-mobility CdSe films. Mild annealing at only 250 °C was sufficient to deliver an electron mobility of 210 cm2/V.s, which exceeds the best reported values for solution-processed inorganic semiconductor. Annealing NC films at 300 °C results in devices with FET mobility above 300 cm2/V.s.
Soldering various materials with novel chalcogenidometallates.
The molecular solders functioned not only for nanoscale but also for mesoscale grains. The solder effect was used to consolidate microparticles into 3D blocks cast with a mold. Chalcogenidometallate solders were also used for bonding metal chalcogenide crystals under modest pressures and temperatures.
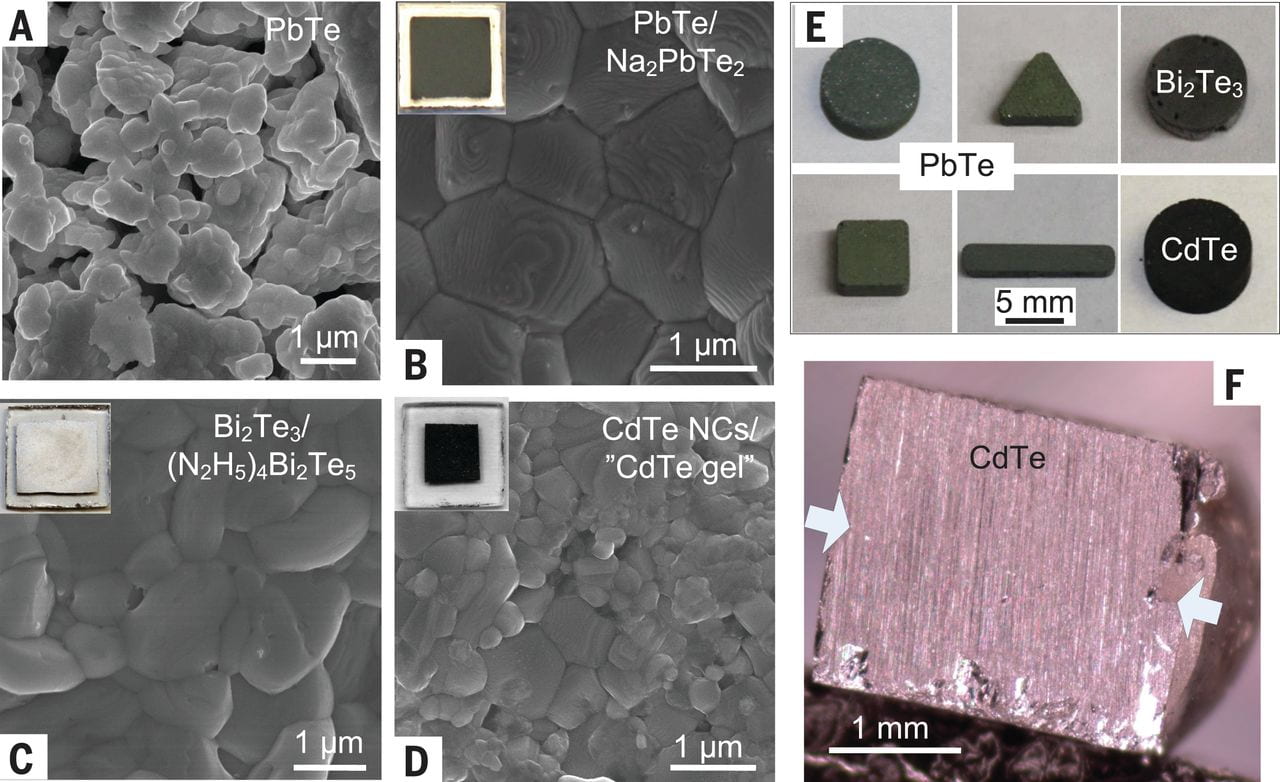
Charge Transport in Arrays of QDs
To employ colloidal nanocrystals in electronic and optoelectronic devices (e.g., in solar cells, light-emitting diodes, and radiation detectors), it is necessary to establish efficient electronic communication between individual nanocrystals. The conduction in nanocrystal solids involves charge transfer between individual nanocrystals via quantized energy states.
Surface ligands with long hydrocarbon chains, used for nanocrystal synthesis, form highly insulating interparticle barriers and result in a very weak exchange coupling between the nanocrystals. Charge transport can be improved by replacing bulky ligands with smaller capping molecules, such as pyridine or n-butylamine, so that the electronic coupling between adjacent nanocrystals increases.
Also, chemical treatments of nanocrystal solids with dilute solutions of hydrazine, methylamine, or 1,2-ethanedithiol can significantly improve carrier mobility, up to about 1 cm2V–1s–1. Unfortunately, such small linking molecules are volatile and susceptible to oxidation, imparting instabilities in the electronic properties. Our group has proposed an entirely new chemical approach for designing electronic materials from colloidal nanocrystals.
Colloidal Nanocrystals with Molecular Metal Chalcogenide Surface Ligands
Spin-coated arrays of CdSe nanocrystals capped with In2Se44– molecular metal chalcogenide complex (MCC) ligands exhibited electron mobility higher than 15 cm2V–1s–1 , which was at least one order of magnitude higher than the carrier mobility in best solution-processed organic and nanocrystal-based devices. Most probably, no other approach could provide a dense film of a direct gap semiconductor with comparable carrier mobility via a simple low-temperature solution-based route. Moreover, our high mobility nanocrystal solids preserved the properties of quantum-confined semiconductors. High carrier mobility, combined with size-tunable electronic structure makes MCC-capped nanocrystals very attractive for printable electronics, photovoltaics, and photodetector applications.
Our group has recently employed lead and bismuth chalcogenide nanocrystals capped with Sb2Te74– MCCs as soluble building blocks for nanostructured thermoelectric materials. Properly designed MCC bridges between nanocrystals can provide high electron mobility and very low thermal conductivity suppressed by the interfacial phonon scattering, which is the combination required for high thermoelectric figures of merit (ZTs). Thermoelectric properties of our nano-composite materials (ZT = 0.7 and 0.6 at 300K and 523K, respectively) compared favorably to all previously reported solution-processed thermoelectric materials, with the potential for further improvements.
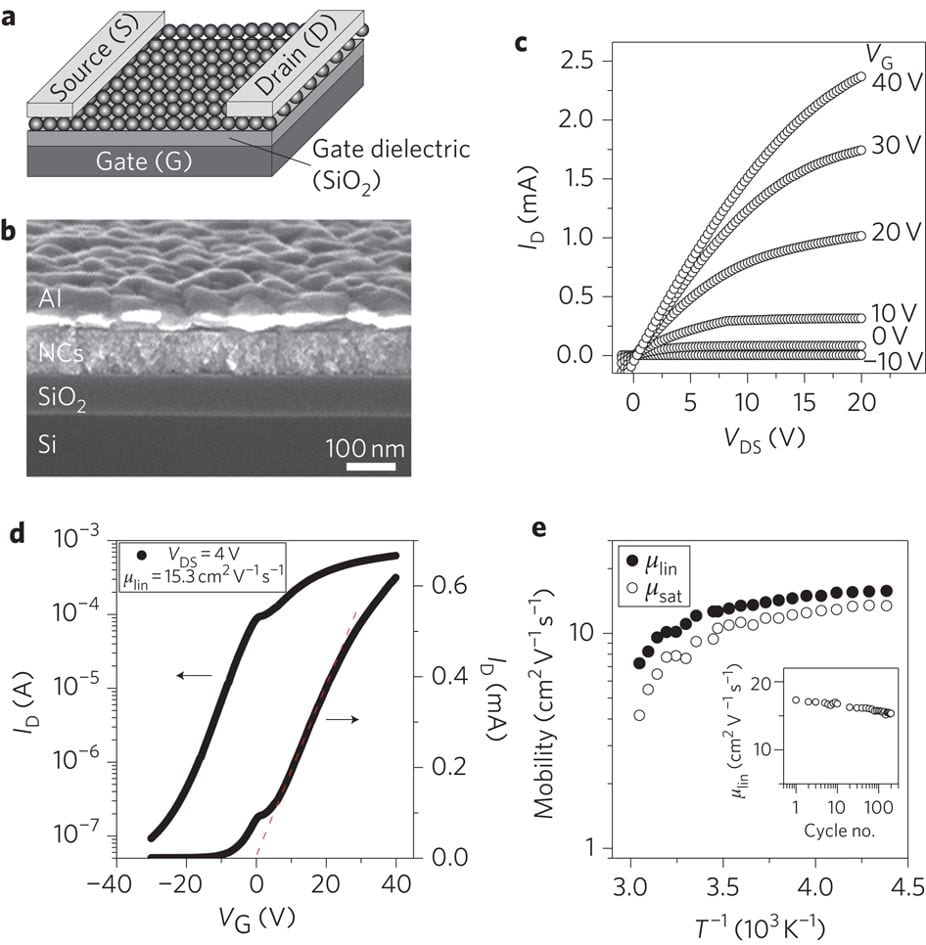
Field-effect transistors.
Field-effect transistors using In2Se42−-capped CdSe nanocrystals.
Colloidal Nanoplatelet Assemblies Enable Rapid Energy Transfer
Colloidal nanoplatelets (NPLs) can be made to stack along the direction of quantum confinement. By combining NPL populations of different thicknesses it is possible to observe energy transfer processes such as FRET between NPLS within this stack. A remarkable property of NPL assemblies is that the timescale of energy transfer can be extremely fast (~10 ns). This process is so fast that it can even outcompete Auger recombination in this system by an order of magnitude. Such properties may potentially be advantageous for a range of optoelectronic applications, including lasing.
FRET within assemblies of stacked NPLs can be so fast as to outcompete Auger recombination.
