Self-Assembly
Self-Assembly of Colloidal Nanocrystals into Ordered Superstructures
Recent studies have demonstrated enormous structural diversity in multicomponent nanoparticle assemblies, leading to a multitude of complex phases combining semiconducting, metallic, and magnetic nanocrystals into long-range ordered binary nanocrystal superlattices. Chemical methods developed over the past two decades enable preparation of colloidal nanocrystals with uniform size and shape. These Brownian objects readily order into superlattices. Recently, the range of accessible inorganic cores and tunable surface chemistries dramatically increased, expanding the set of nanocrystal arrangements experimentally attainable.
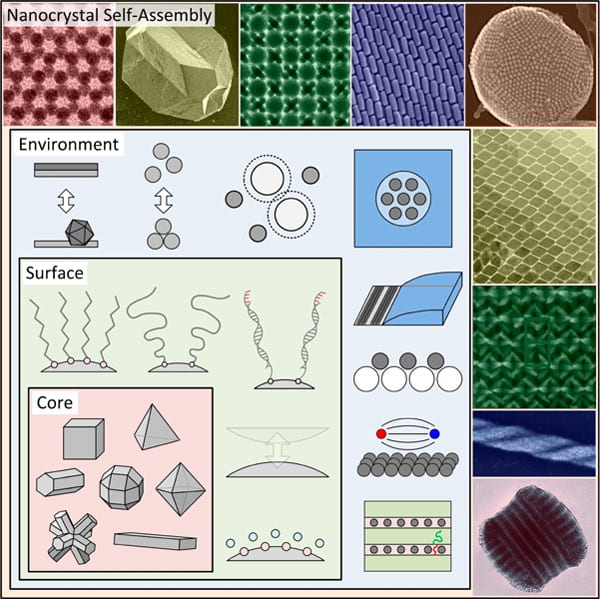
Nanocrystal self-assembly is a process that involves control over several length scales. The nanocrystal core (typically 1−100 nm across) is surrounded by a layer of surface ligands (with length typically between 1 nm and up to tens of nanometers). The assembly environment can be used to control interparticle interactions and impart geometric constraints with characteristic length scale exceeding nanocrystal size. The resulting superstructures are typically produced with domain size falling between 1 μm and several millimeters.
Nanoparticles have been found to form a very rich set of binary phases.

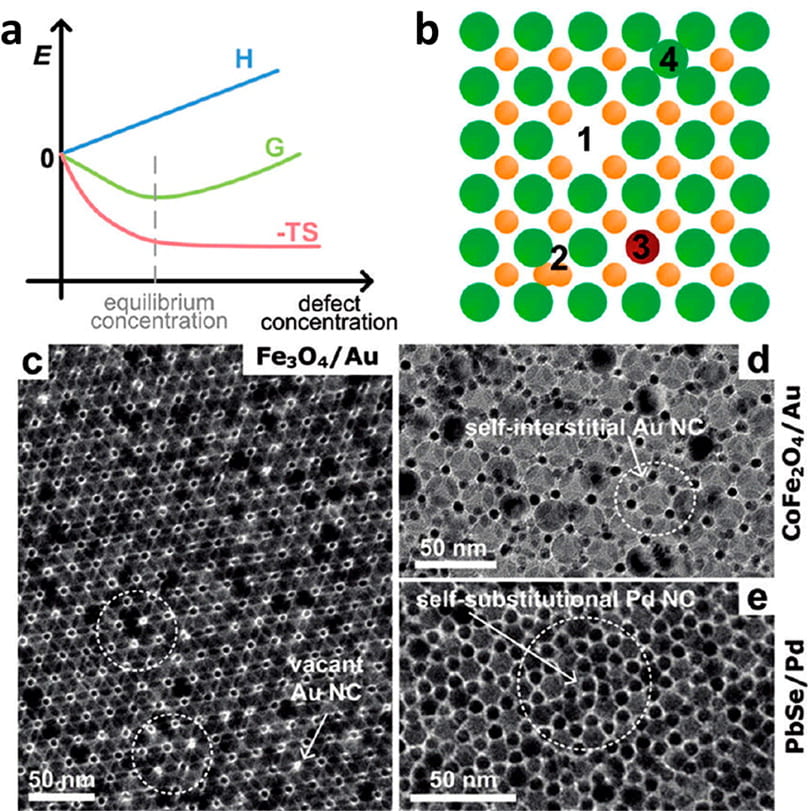
Defects in nanocrystal superlattices.
Through a combination of synthesis, structural characterization, and data processing it is possible to experimentally evaluate competing models of structural packing. In this case the data showed that the optimal packing model (OPM) described the structure of single component assemblies.
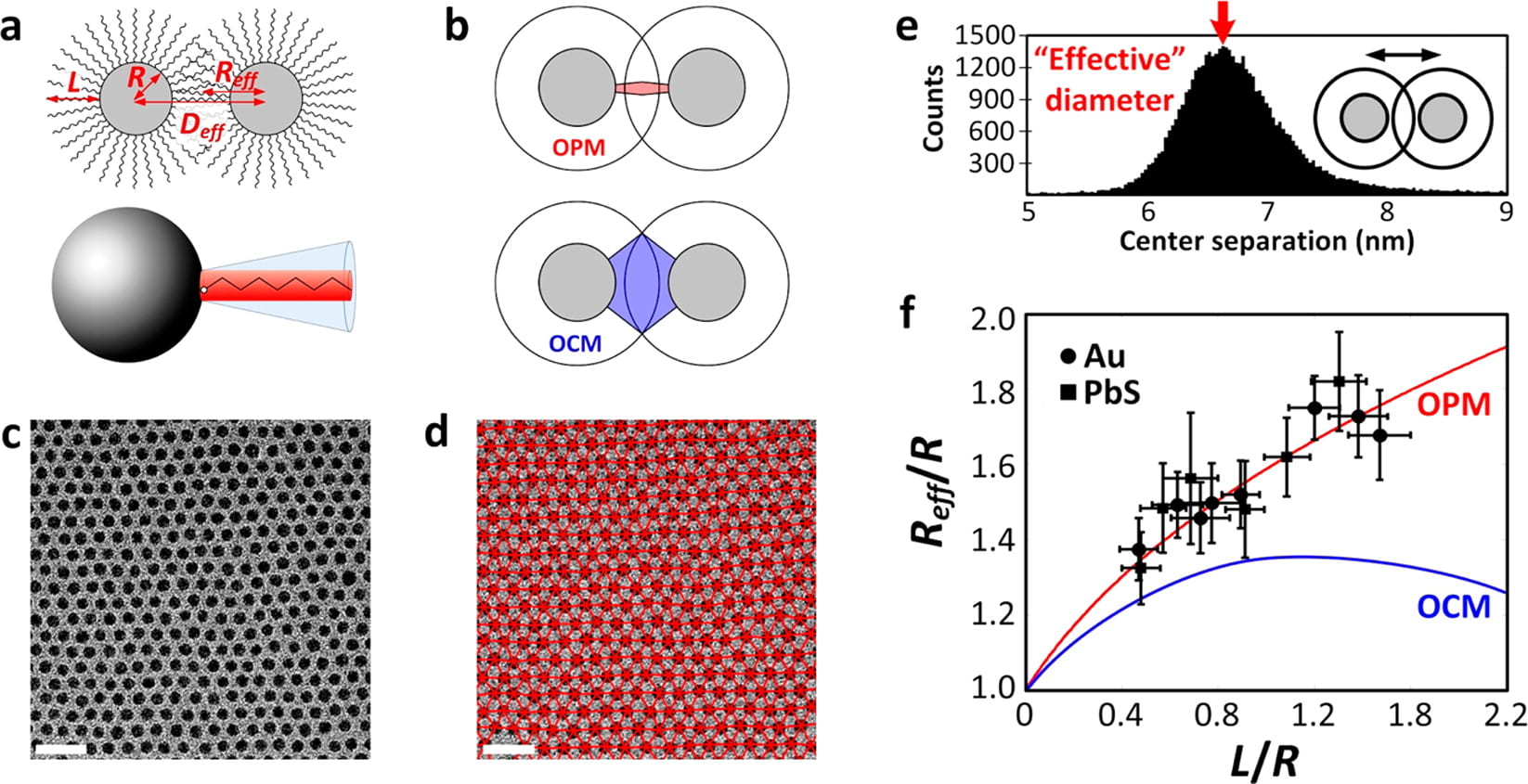

Structural diversity of binary nanocrystal superlattices and low packing density predictions for most observed phases.
By systematically studying the formation of binary superlattices we can formulate new hypotheses about the mechanism that favors the formation of specific phases. An interesting conjecture is that the symmetry of the intermediate liquid can facilitate nucleation of phases with unit cells having a higher degree of icosahedral order.

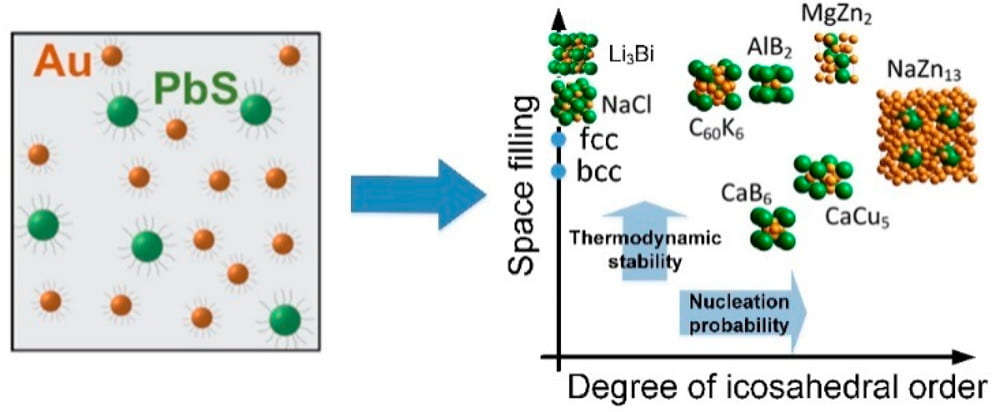

(a) Schematic demonstration of the orbifold topological model (OTM). In OTM, the ligand corona of a particle can be distorted by vortices where the orientation of the ligands points away from the point of contact as shown by the vector field (orange arrows) in the middle panel. This distortion in the ligand corona in turn allows a neighboring particle to approach at a distance . (b) Different possible skyrmion textures for different numbers of vortices. (c) Packing fraction analysis for Li3Bi showing the appearance of a new OTM branch at higher density than the HS branch; red ● denote experimentally measured results.